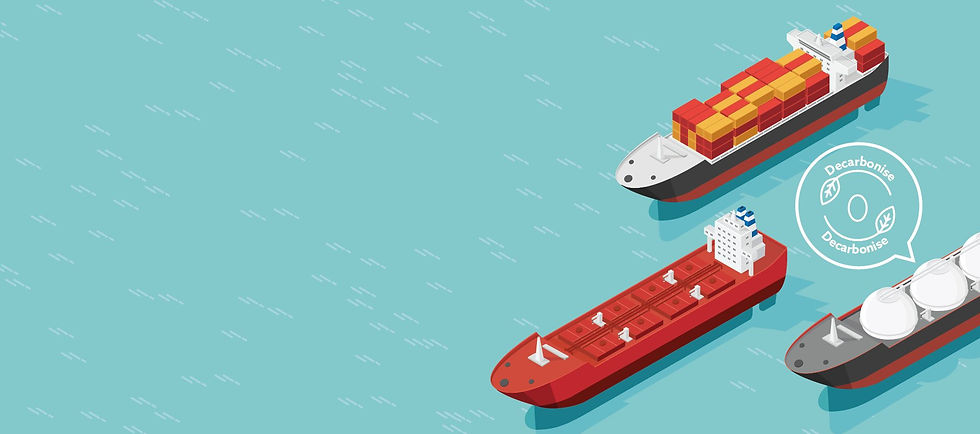
Insights
A feasible pathway to greener, faster and cheaper maritime decarbonisation
13 August 2024
The four most obvious alternatives to fossil fuels for maritime decarbonisation are:
Hydrogen: zero emissions when produced from renewable sources (green H2)
Ammonia (NH3): recognised for its potential as a carbon-free fuel with a relatively low production cost
Methanol (CH3OH): a relatively safe liquid fuel capable of powering marine engines using existing vessel infrastructure
Electric battery systems: a clean and mature energy solution with the capability to reduce emissions and noise pollution, however currently limited to short voyages
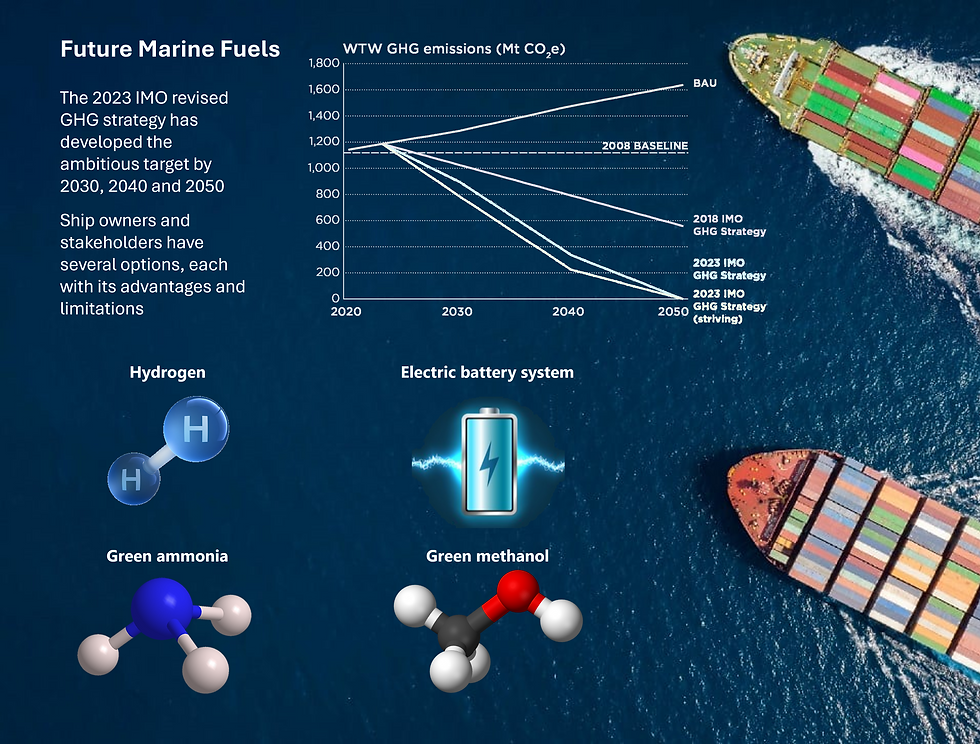
Four most obvious options for maritime decarbonisation
A wide adoption of H2? Probably not
Hydrogen can be an alternative fuel for vessel propulsion using either fuel cells (FC) or internal combustion engines, with well-to-wake efficiencies rated at 30-35% and 20-25%, respectively.
Commercial utilisation of hydrogen in marine vessels has been largely limited to small ships such as the Hydrogenesis Passenger Ferry operating in Bristol, UK, and the newly launched hydrogen-powered commercial passenger ferry in San Francisco Bay. Although larger ships, including container ships, equipped with hydrogen fuel cells are being commercialised, e.g., the MF Hydra ferry in Norway, the H2 Barge 2 in the Netherlands, as well as the announced SeaShuttle project in India, all of these are hybrid vessels: the MF Hydra and H2 Barge run on hydrogen fuel cells and batteries, and the SeaShuttle runs on hydrogen fuel cells and a diesel engine.
The barriers to H2 implementation are substantial
“The storage capacity of hydrogen, in either liquid or compressed states, will be a challenge for certain ship types”. – European Maritime Safety Agency [1]
Although hydrogen has a high energy density per weight, it has a very low volumetric energy density, therefore requiring significant storage space onboard a vessel. Hydrogen can be stored as a compressed gas at up to 700bar but would still suffer from low storage densities. Practically it needs to be liquified (LH2) under extremely low temperatures (-253°C) requiring specialist storage systems. The liquification process will also waste more than 1/3 of the energy from an already inefficient energy carrier.

H2, methanol and ammonia, a quick comparison of characteristics
Safety is another challenge. H2 is the lightest of all atoms, making it hard to contain, and it can embrittle materials that would be safe to use with natural gas. Additionally, there are issues with hydrogen as a marine fuel related to hydrogen’s flammability range, leakage, flame speed, and detonation/ deflagration characteristics.
Economic viability remains a concern for hydrogen. The high initial investment and operational costs still require substantial cost reduction to compete with conventional fuels, some of which could come from increased economies of scale that could be achieved in bulk transportation and storage.
“Vessels plying short-sea routes – primarily coastal vessels – have the potential to adopt hydrogen as a fuel because their frequency of port calls and bunkering would support lower bunker capacities once hydrogen-bunkering infrastructure becomes available. In long-range shipping, the total cost of ownership (TCO) for hydrogen-fuelled vessels in principle remains as a barrier. The example cases of ferry Ro-Pax and Ro-Ro vessels present a TCO for green hydrogen that is about 3 times higher than vessels powered by conventional (fossil) fuels in 2030, and about 20-30% higher TCO in 2050.” – European Maritime Safety Agency [1]
For hydrogen to contribute to the shipping industry's green transition, infrastructure to support the distribution, storage, and supply of hydrogen fuel would be crucial. However, this infrastructure does not yet exist. While some argue that LNG terminals could be adapted to handle hydrogen, we believe there are significant economic, logistical, practical and safety challenges involved in repurposing these terminals for LH2 (or ammonia).
Methanol has a head start
Methanol, like conventional fuels, is a hydrocarbon and a liquid. Today, there are dual-fuel engines (e.g., MAN 49/60DF, Wärtsilä 32 methanol engine) that can run traditional fuels and methanol.
Compared to other alternatives, methanol’s features make it particularly attractive for the maritime sector.
It is non-cryogenic, liquid at ambient temperatures and does not require refrigeration or specific and expensive materials for tanks or pipes
Its full miscibility in water allows storage in vessels with double hulls, rapidly diluting any spills and minimising environmental impact.
Appropriate storage and handling technologies and expertise exist, and bunkering would be comparatively easy to implement.
Methanol has gained an advantage over ammonia in maritime applications over the recent years, largely because regulations for using methanol as a fuel are already in place. The adoption of the IMO interim guidelines for ships using methyl or ethyl alcohol as fuel (MSC.1/Circ.1621) has encouraged shipowners to order methanol-fuelled ships, with major orders for dual-fuel engine vessels being placed by Maersk [2].

There are currently 257 confirmed methanol fuelled ships [3]
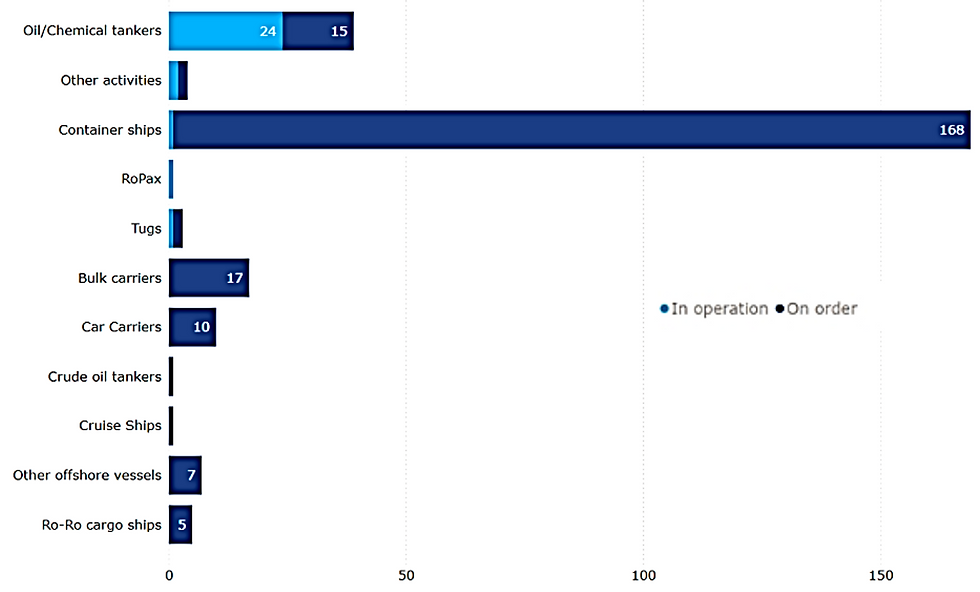
Methanol fuelled fleet by vessel type [3]
Being one of the top five chemical commodities shipped globally, methanol enjoys well-established infrastructure, ensuring widespread availability. Additional terminals for fuelling vessels would however be required.
The long-term uncertainty of green methanol
To be a green fuel, methanol must be made from non-fossil feedstocks, i.e., biomethanol or e-methanol.
Biomethanol can be made from various biomass-based feedstocks, including organic waste from agricultural and domestic sources or the forestry industry. However, our recent study on biomass availability concluded that, unless there is a significant increase in high-energy feedstocks on degraded land (i.e., land that is unsuitable for crop production) on a global scale coupled with proven pre-treatment/ conversion technologies that maximise carbon efficiency and minimise the required feedstock, the amount of biomass is unlikely to be enough to meet the demand for aviation and maritime fuel.
Additionally, we anticipate that biomass sustainability will become a more controversial issue as demand and incentives increase.

Biomethanol vs. e-methanol production pathways
In theory, the long-term methanol supply would come from e-methanol, which requires a steady supply of carbon dioxide. But to achieve carbon neutrality or carbon negative, we only have two options: biogenic CO2 or direct air capture (DAC) – neither of which are without significant techno-economic issues. The exact challenges related to both are explained in detail in two previous articles: "Sourcing and use of biogenic CO2 - and associated challenges" and "The role of Direct Air Capture (DAC) in battling climate change". Overall, the long-term availability and cost competitiveness of green methanol creates a big question mark to its longevity in the maritime sector.
Fuel cells powered by methanol, also known as direct methanol fuel cells (DMFCs), are a theoretical option. The Danish company Blue World Technologies is currently producing methanol fuel cells for the shipping industry. They are initially focusing on replacing diesel-powered generation with methanol fuel cells for auxiliary power units (APUs) on ships. In the future, the company also plans to provide fuel cells for ship propulsion.
Ammonia avoids the need for a carbon source
Using ammonia as a fuel is not currently legal. The IGC code of the International Maritime Organization forbids the use of ammonia as a fuel due to its toxicity. Despite this, ammonia is attractive as it is made from hydrogen and nitrogen – it does not contain any carbon therefore does not require a carbon source. However, there are potential drawbacks to consider as well.
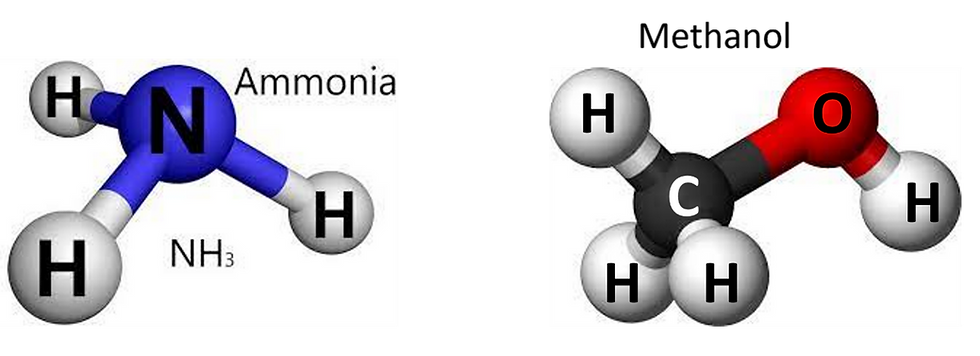
Ammonia needs pilot fuel for ignition since ammonia's high auto-ignition temperature makes it difficult to burn readily or to sustain combustion. Two-stroke ammonia ICEs need around 5-20% pilot fuel, while four-stroke engines may require as much as 30% [4].
As a result, ammonia's zero-emission potential is undermined by using fossil fuel as pilot fuel, which emits carbon dioxide when combusted.

Well-to-wake GHG emission profiles [5]
Although innovations for ammonia-fuelled ships without pilot fuel are underway, such as the ammonia-powered gas turbine proposed by South Korean company Hanwha Group [6]. Unfortunately, they are in the concept stage and still far away from commercialisation.
E-ammonia is cheaper to produce
E-ammonia, which is produced by combining green hydrogen and nitrogen (a similar principle to e-methanol), is not yet being produced at a large commercial scale. But since there is no need for a CO2 source and nitrogen is easily available (comprising 78% of the atmosphere), the cost of producing e-ammonia will be significantly lower than that of e-methanol.
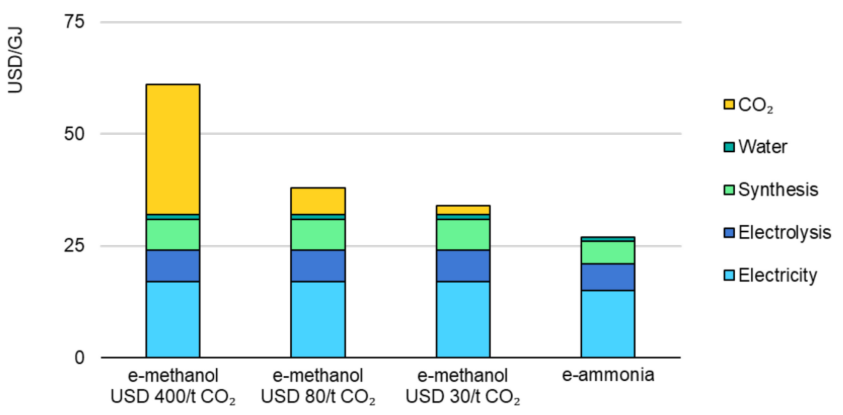
Levelised cost of e-ammonia and e-methanol at different capture cost for CO2 feedstock [7]
Notes: CO2 capture costs USD $30/t - CO2 from high-concentration sources; USD $80/t - CO2 from flue gases; USD $400/t - CO2 from direct air capture
The Chinese energy company Envision is now capable of producing green ammonia at the same cost as grey ammonia. They have achieved this by creating a reliable and efficient renewable energy system. Envision directly connects wind energy with an electrolyser, which brings down the cost of wind energy to around 3 cents/kWh, and are currently in the process of building a 1.52 million ton-per-year green ammonia facility [8].
The combination of “intolerable” risks and high OPEX may be a deal breaker
There are challenges with ammonia. It has a lower energy density than methanol, it needs to be kept under pressure and it is a challenge to reliably combust.
However, the most serious challenge is that ammonia is very toxic. When ammonia gas is inhaled it can be fatal. When spilled, ammonia dissolves in water and forms ammonium hydroxide, which is highly toxic to marine life. When it hits surface water, for example following a vessel collision, it reacts with condensation in the air to form a heavy, toxic mist that is very difficult to clear.
There are also issues with ammonia combustion. Burning ammonia produces NOx and N₂O (nitrous oxide - laughing gas). NOx emissions contributing to air pollution. N2O, a greenhouse gas, has 300 times the global warming potential than CO2. Furthermore, incomplete combustion can lead to toxic atmospheric ammonia emissions. For ammonia to succeed as a green fuel, apart from strong safety standards, it will also need technologies such as SCR (Selective Catalytic Reactors - currently commercially available), which will add additional CAPEX and OPEX.
Methanol is more expensive to produce, but ammonia is more expensive to handle
Both ammonia and methanol are hazardous chemicals, but ammonia is toxic at much lower concentrations. It is corrosive which requires additional costs for corrosion-resistant tanks, and safety measures such as spaced-out storage, double piping, leak detectors, and dedicated ventilation systems. These safety precautions make it more expensive to use ammonia as a fuel for ships.
Despite these challenges, strong global initiatives are exploring the possibility of using ammonia as a marine fuel. A more efficient approach could be direct ammonia fuel cells (DAFCs), an emerging technology that can directly use ammonia to generate reliable, clean power without combustion or thermal cracking. However, DAFCs are currently at an early stage of development (TRL 3-4). The European Union project Ship FC is one of the ongoing initiatives focusing on this technology.
Electrification – a better and potentially cheaper option that is currently overlooked?
The most direct and efficient way to use green electricity in ships is through batteries. Electric-battery systems have the highest energy efficiency, ranging from 75-85%+ depending on transmission and charging losses. This makes them the lowest total cost of ownership low-carbon alternative to fossil-based fuels.
However, due to their heavy weight, large volume, and limitations in power supply and speed, conventional thinking suggests that battery-electric propulsion would only be suitable for ferries or smaller vehicle-and-passenger ships, whereas containerships carrying up to 24,000 twenty-foot (TEU) containers would require alternative fuels as energy carriers.
Multiple recent studies investigated the role of electrification in the maritime industry in considerable detail have argued otherwise. Researchers from the University of California at Berkeley and the US Department of Energy’s Lawrence Berkeley National Laboratory [9] have determined that it is economically feasible to electrify over 40% of global container ship traffic within the 2020s. According to their models, the total cost of propulsion for a battery-electric ship is lower than that of an HFO-fuelled vessel for ships larger than 8,000 TEU and voyages of less than 1,000 km.
When factoring in environmental and human health costs from emissions, the cost-effective range of battery-propelled container ships expands to 5,000 km for all ship sizes. Additionally, with projected improvements in battery energy density and lower overall costs, the cost-effective range of battery-driven ships increases to around 3,000 km for all ship classes. Considering environmental costs, batteries’ energy density improvements and cost reduction, the authors concluded that the range expands to 6,500 km for smaller ships and up to 12,000 km for the largest ship classes.
In a report published in 2024 by the Institute of Electric Power Systems at Leibniz Universität Hannover, Germany [10], it was concluded that, with the inclusion of a carbon tax and optimistic forecasts for battery development, battery-powered solutions are more economically competitive than conventional diesel engines for voyages of up to 2,500 km, regardless of the size of the vessel. The study also found that the use of battery-electric propulsion systems is not limited by vessel size but rather by the distance of the voyage. While distances of up to 15,000 km are technically feasible, economic constraints limit the practical application to a maximum of 10,000 km.
Indeed, the sizes of installed marine battery systems are consistently increasing, with the Yara Birkeland becoming the first battery-electric container ship to enter commercial operation in 2022 [11]. In 2023, the Chinese operator Cosco Shipping Heavy Industry launched a 700 TEU battery-powered electric container ship on the Yangtze River. This 10,000-ton ship is powered by completely swappable batteries and can travel the 600-mile inland route without emissions [12].
It is safe to expect faster breakthroughs in battery technologies (e.g., energy density, stack and cell costs) than major breakthroughs needed for other alternatives, considering the intensity of ongoing R&D activities and investments worldwide. An example of this is the semi-solid state "condensed" battery introduced by CATL in 2023, which has an energy density of 500 Wh/kg (compared to the Tesla Model 3's 260 Wh/kg). This marks just the beginning of advancement in battery energy density [13].
For short-sea shipping, we believe that battery-electric propulsion has not been fully explored as a low-emissions alternative in the marine shipping sector. Its significant potential to reduce emissions, decreasing battery costs, improvements in battery energy densities, the growing availability of low-cost, renewably generated electricity, and its substantial efficiency advantage over e-fuels like green hydrogen and ammonia make electric-battery systems a more practical option for reducing emissions in leisure boating, small commercial ferries, fishing boats, feeder cargos, inland cargos, and regional/coastal cargos - that's a lot of sea-borne emissions!
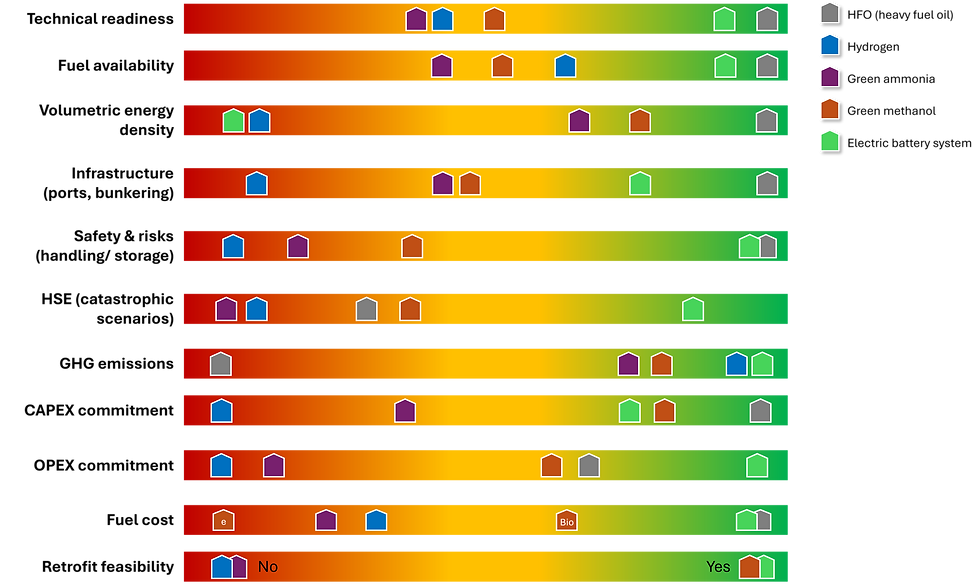
Our assessment of four alternatives (using HFO as a benchmark)
While batteries have numerous advantages, we also recognize there are challenges such as limited range, the need for reliable onshore/offshore power supplies and charging infrastructure, its implementation across diverse vessel types, and safety concerns (e.g., thermal runaway).
What about long distance deep-sea shipping?
Unfortunately, global statistics on deep-sea shipping versus short-sea shipping (which includes inland shipping on rivers and lakes) are not available therefore we were not able to quantify the amount emissions associated with short-sea shipping that can be avoided by electrification. However, the data that is accessible indicates that short-sea shipping is responsible for over 70% of sea transport in the EU – a significant and accessible prize for vessel electrification.
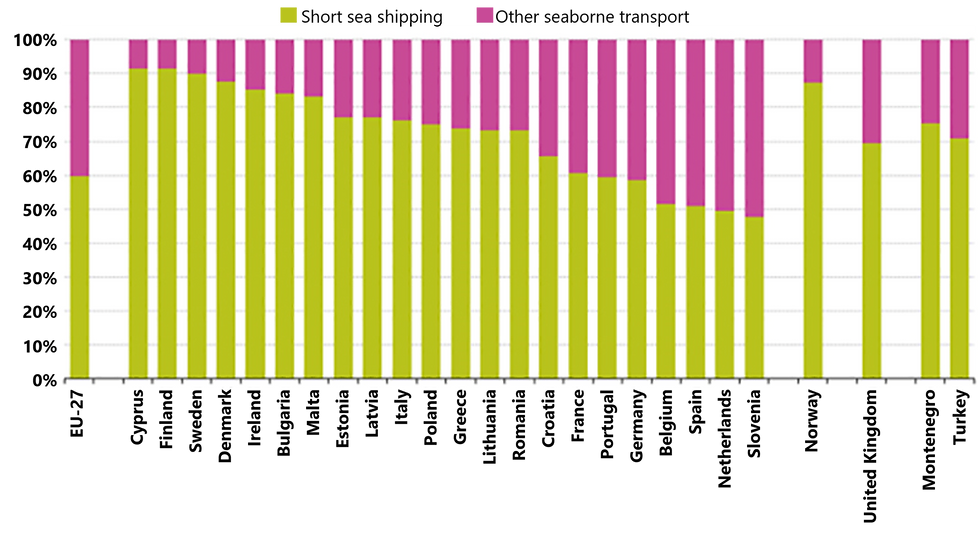
Short Sea Shipping is responsible for more than 70% of sea transport in this region, which consists of the interconnected seas of Eastern Med, the Aegean, the Marmara Sea, the Black Sea and all the rivers that flow into the Black Sea [14]
In the short to medium term, the limited biomass-based feedstock should be fully utilised for biofuels (not burning, as we will explain in a future thought piece). In a positive scenario, we should also expect to see an increase in high-yield feedstocks and better biomass pre-treatment and conversion technologies.

What is biofuels?
In the long term, e-fuels and fuel cells will become available and become cost-competitive, but only when "electrification of everything" is achieved. This will lead to a surplus of cheap green electricity that can be used for secondary purposes, such as e-fuels for the aviation and shipping industries where direct electrification is not possible. Within this scenario, it is clear that vessel electrification has a compelling role to play in maritime decarbonisation, both as an immediately accessible alternative to fossil-based fuels, and as a long-term part of the propulsion energy mix.
References:
[1] Potential of Hydrogen as Fuel for Shipping. EMSA (European Maritime Safety Agency. 31/08/2023 - 4837444
[3] DNV data: https://www.manifoldtimes.com/news/dnv-23-methanol-fueled-ships-registered-in-january-orderbook/
[5] Kongsberg Maritime data: https://safety4sea.com/cm-is-ammonia-the-solution-for-a-carbon-free-shipping/
[7] The Role of E-fuels in Decarbonising Transport. IEA (International Energy Agency). Revised version, January 2024
[9] https://www.nature.com/articles/s41560-022-01065-y
[10] https://www.sciencedirect.com/science/article/pii/S2590174523001630
[11] https://www.yara.com/news-and-media/media-library/press-kits/yara-birkeland-press-kit/
[12] https://interestingengineering.com/transportation/battery-powered-container-ship-china-yangtze-river
[13] https://www.catl.com/en/news/6015.html
[14] https://unctad.org/news/short-sea-shipping-and-energy-transition